Chris Meyer, Past President, Ontario Association of Physics Teachers
chris_meyer1@sympatico.ca
Why explore the idea of polarization?
A good way to learn about static electricity is by exploring things around us: run a comb through your hair, peel different fabrics apart, go down a plastic slide in a playground, there's so many possibilities! Another common one is the rubbed balloon sticking to a wall: an example of a charged and uncharged object interacting that requires some subtle science to explain. The grade 6 science curriculum mentions examples like this but leaves out the scientific tools to properly explain it. The grade 9 curriculum mentions charging by induction and again leaves out the science. Electrical interactions form the conceptual backbone for an understanding of atoms and molecules, electric circuits and fields, and why those annoying bits of Styrofoam keep sticking to our hands! The missing idea from the curriculum is polarization, a concept that explains the attraction between a charged and neutral object. Polarization also serves as a conceptual bridge between the topics of static and current electricity, which are often taught as two distinct topics. The curriculum provides a poor road map for learning about electricity; it will pay us great dividends as teachers to do a better job of this, so let's explore polarization! I want to share with you
two lessons for grade 9 science that focus on this important electrical concept.
Evidence for polarization
Science starts with observations: we see something unusual that doesn't fit into our understanding. If we've only learned about the “
law of electric charges”, we have no simple way to explain this observation experiment:
Law of electric charges for elementary particles. This law is often introduced in science textbooks but with part of its name missing, the “elementary particles” part. The law works great if you are working with individual electrons and protons but becomes confusing when working with everyday objects. Unfortunately, the law can't be applied directly to macroscopic objects like balloons and walls. Charged and neutral objects will attract, which will throw off most student attempts to apply or make sense of this law.
The truth is out there
It is our job as scientists to search for evidence that helps us understand how this interaction works. Let's come up with a few different ideas which we will test as hypotheses:
- They attract because the wall becomes charged.
- They attract because electrons in the wall get pushed around.
Both of these are quite plausible ideas that must be tested! Here are some testing experiments that might eliminate an unreliable hypothesis.
- Check if there is attraction without the objects touching.
- Check if the wall becomes charged.
- Check if charges in the wall move around.
Types of experiments. It is really helpful to understand the different ways that experiments are used in science. Experiments can fall into three categories depending on their role in the development of scientific knowledge:
- Observation experiment: an experiment that provides evidence for new phenomena and kickstarts the search for new scientific ideas or explanations.
- Testing experiment: an experiment that discriminates between or eliminates rival hypotheses in an attempt to develop a new scientific explanation.
- Application experiment: an experiment that uses a well-tested idea to predict what happens in a novel situation or to refine a well-tested explanation.
Let's practice making predictions: time for some good ol’ hypothetico-deductive reasoning! Here is the chart we use to help organize this thinking for the first testing experiment.
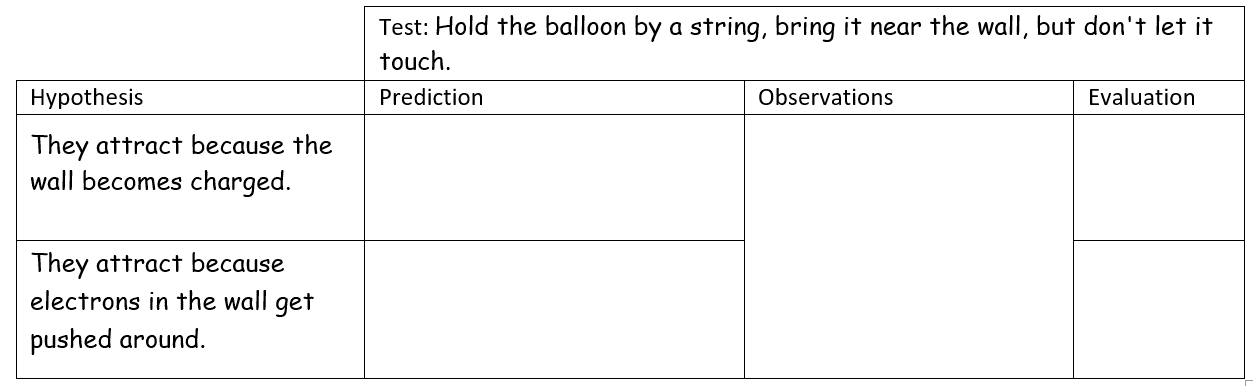
Now it's your turn. Produce a prediction using
each hypothesis for this one experiment. The thinking process goes like this: suppose the first hypothesis is correct, describe what we would observe in this experiment. Repeat this process for the second hypothesis. Once you are ready, watch the testing experiment video below.
Which hypothesis do your observations support or refute? Here is a related testing experiment that I have included in case students come up with this idea:
The first hypothesis is now definitely refuted! However, we need a more stringent test for our second hypothesis: let's look for evidence that electrons move in the neutral object. To find this evidence, let's replace the wall with a metal rod, which allows electrons to move easily; and replace the balloon with a PVC pipe, which can hold a stronger charge. We will hold the negatively charged pipe near one end of the rod. Take a moment and make a prediction: what will we observe when we bring a string near the middle and end of the metal rod? Let's watch the video and find out!
Does the evidence support or refute our second hypothesis? Here we can almost see the phenomenon of polarization. Isn’t this fun?
Seeing the invisible
Electrons are awfully small and hard to see. An important strategy for learning science is creating visual representations for things that are abstract or invisible. Let's do this for polarization. We can start by creating a model for the shifting of the electrons in the metal rod using coloured sheets of translucent plastic. A red piece represents the outer electrons of the metal atoms. A blue piece represents the rest of the metal atoms (minus the outer electrons). When the charged pipe is far from the metal rod, the two coloured pieces overlap perfectly:
Defining polarization: it took a while!
When the charged pipe is brought near the metal rod, the rod becomes polarized. Now we're ready to define this novel scientific idea: electrons in the neutral object shift due to the charged object nearby. Think about the amount of work that went into creating this definition. Science requires careful investigation and a fair bit of patience! We shortchange our students when we present a scientific idea or definition without much evidence or justification. Let's return to our coloured plastic model:
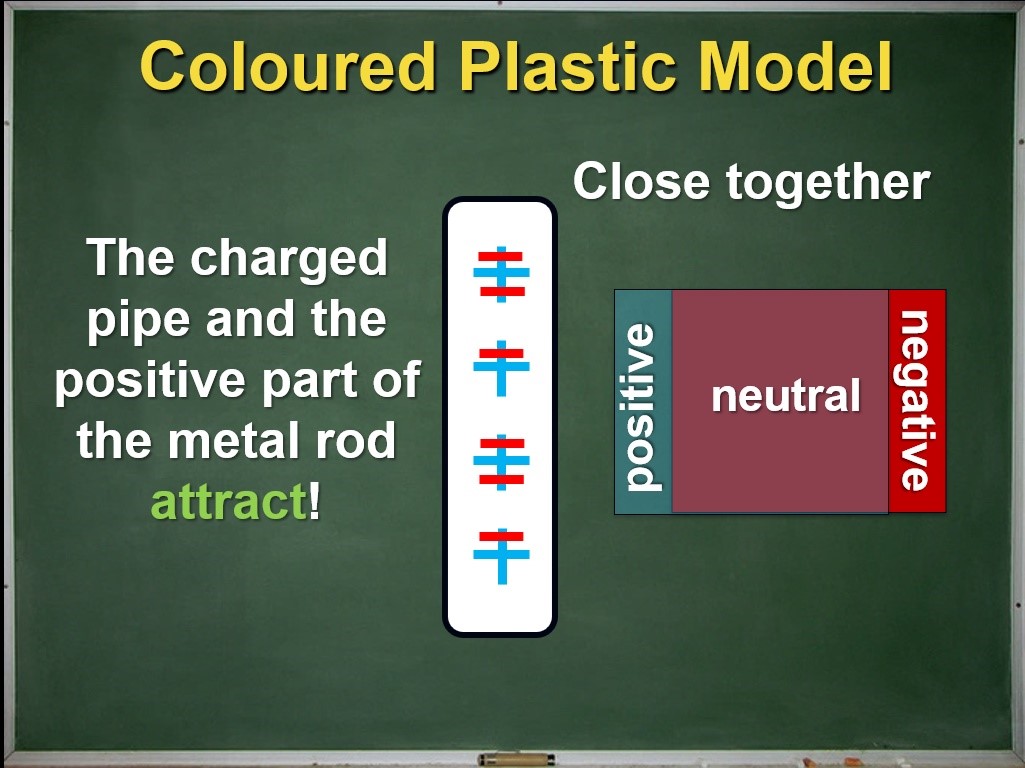
Does this model match our observations with the string? Go back and watch the video if you are not sure. Even though the metal rod is still neutral (net charge of zero) overall, its opposite ends now have opposite charges while its middle region is electrically neutral.
Charge diagrams for polarization
Now we can refine our visual representation by replacing the two colours of plastic with symbols for the positive and negative charges in solid matter. Our convention here is that the red “-” symbol represents the outermost electrons of an atom and the blue “+” symbol represents the rest of the atom (sometimes known as the positively charged ionic core).
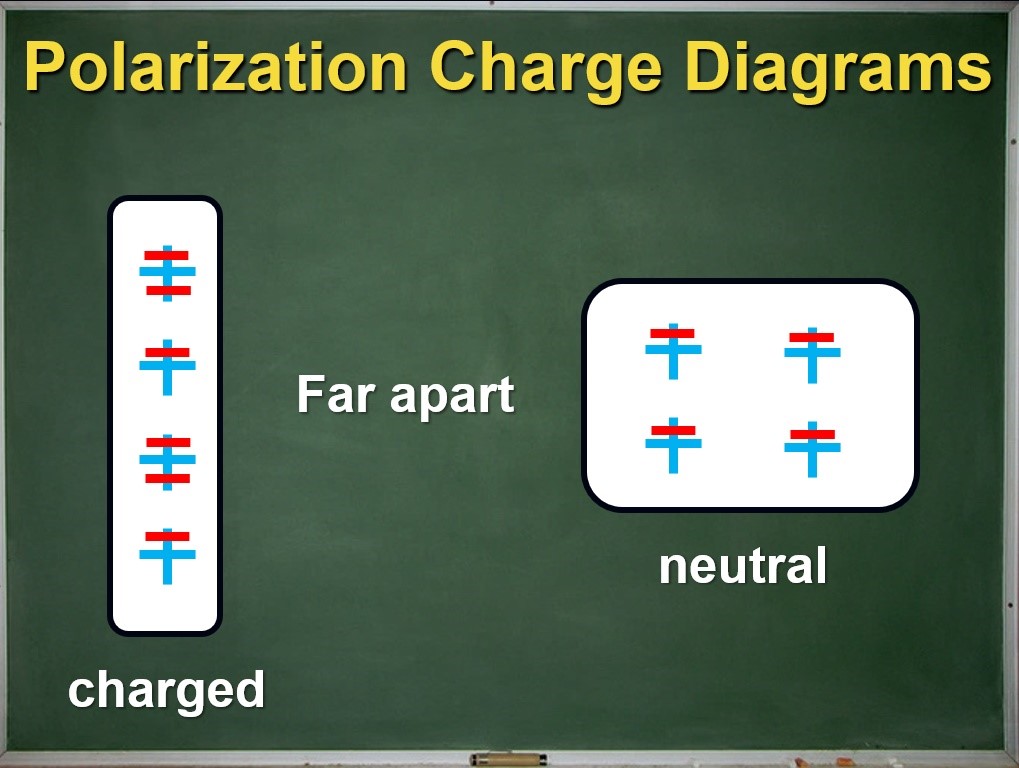
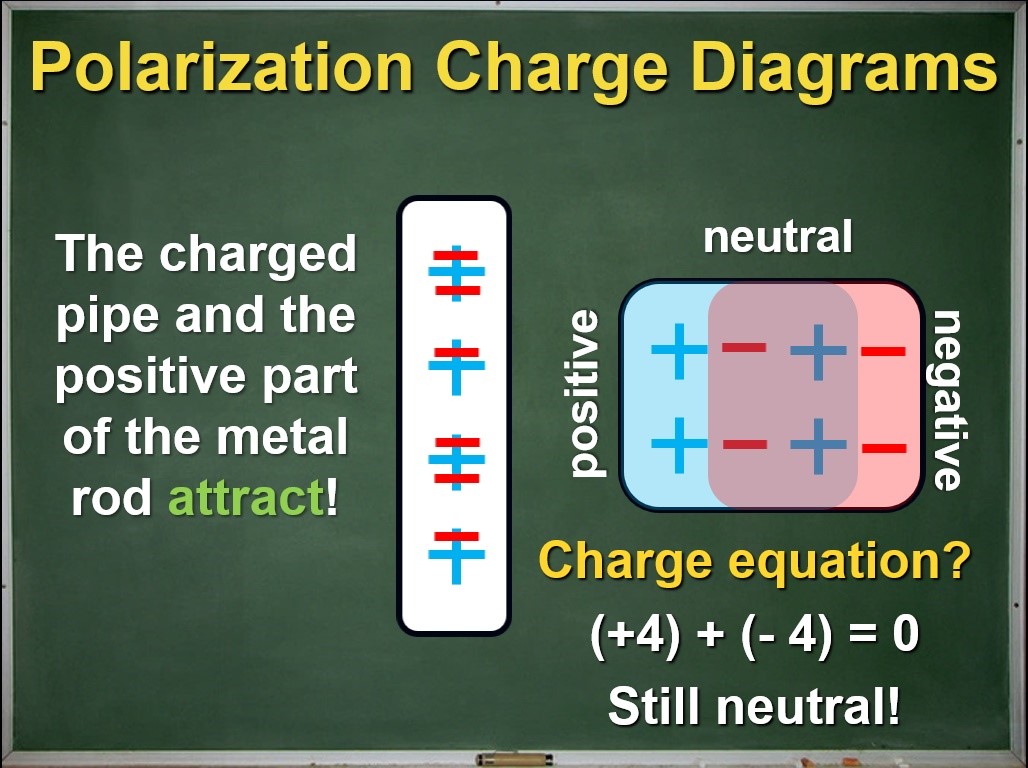
After reminding students that the ionic core of an atom is much heavier than its outer electrons, the charge diagrams can reinforce the idea that the electrons shift during the process of polarization, but the rest of the atom does not. A quick check shows us that the net charge of the metal rod is still zero. Now we have a clear physical model for what happens inside a neutral object during polarization.
The importance of physical models
In science, a model is a set of ideas and tools that help us explain or predict how something works. A good model should be based on clear, well-tested, and broadly applicable ideas. I think the model for polarization presented above in its charge diagram representation has these characteristics. I am fairly confident this model is much better than what I used to teach, and what is commonly found in textbooks and on the interwebs.
Problems with common charge diagrams
Diagrams such as the ones shown here illustrate regions within an object where the net charge is not zero.
https://www.physicsclassroom.com/class/estatics/Lesson-2/Charging-by-Induction This is conceptually challenging for students and is often not emphasized: the pluses and minuses in these diagrams do not directly represent charged particles, they represent regions of net charge. Students begin to interpret these diagrams in a simpler way, assuming that the plus and minus symbols represent separate particles that are able to move. This undermines an important physical concept for solid matter: the atoms (or the positively charged ionic cores) are fixed in place and cannot move — it’s a solid!
But wait, there's more!
In our second lesson on polarization, students watch an application experiment. Two spheres hanging from strings, one sphere is a conductor while the other is an insulator. A negatively charged PVC pipe is brought near the two spheres. Will there be any difference in the interaction between the spheres and the pipe? Make your prediction!
Most of my students will predict this one correctly because we have a good model for conductors versus insulators. Here we can't see the difference in the shifting of the electrons, but we can deduce that there is a greater shift in the conductor. As a result of this observation, we refine our charge diagrams for insulators to highlight the idea that the outer electrons are localized (with an oval outline), meaning very tightly bound to the rest of the atom and shifts less.
The great big sea
We also refine our understanding of the polarization of conductors. Electrons in a conductor are mobile, meaning they can easily move around the material. This motivates the introduction of an important idea called the sea of electrons: the mobile electrons in a conductor tend to move as a group through the material, much like water flowing along a river.
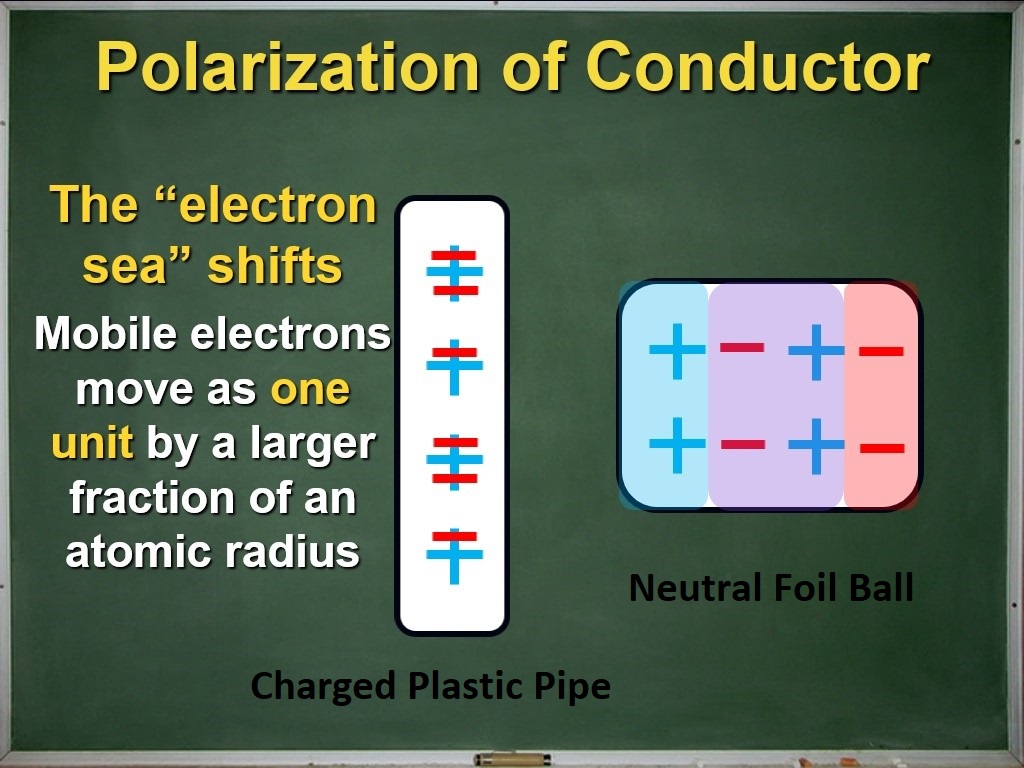
Our careful work building a physical model for polarization has borne great fruit: we now have a physical model for current electricity which we can show by continuously sliding the red film across the stationary blue film! This also helps to displace an important misconception or naive understanding: a neutral piece of metal (a wire, for example) is not empty and waiting to be filled with electrons after contacting a charged object or a battery in an electric circuit; it is already “full,” which is what neutral means! It also lays the foundation for understanding why electrons are not “used up” when they pass through a load in a circuit.
An investment in science learning
Our physical model for polarization creates a bridge between the topics of static and current electricity. Aside from having the same “last name”, which might lead some to suspect a relationship between these two topics, they are often taught using different conceptual frameworks. The amazing thing about science is how a small quantity of powerful ideas can explain a wealth of phenomena. It is important that our science teaching helps students to connect these dots so that rather than learning disconnected facts about the world, they are learning powerful models that highlight deep connections. Unfortunately, the curriculum does not help us do this. So extra-curricular excursions and tweaks, like this one, are necessary for good scientific learning and will help students when they tackle more advanced ideas in their senior science courses.
Resources
An electronic version of the
two grade 9 lessons, the accompanying PowerPoint, and various videos are all available
here.
Tags: Electricity, Pedagogy